AEROBIC AND ANAEROBIC BIOTREATMENT OF OLIVE OIL MILL WASTE WATER
Hashwa, Fuad & Mhanna, Elias
Lebanese American University, Byblos, Lebanon
Abstract
Olive Oil Mill Wastewater (OMW), known in Lebanon as Zibar, is one of the two by-products obtained during olive oil extraction. OMW represents a serious environmental pollution problem especially for underground and surface water. Aerobic and anaerobic OMW bio-treatment processes were developed and improved and showed promising success. A bacterial mixture of ten strains (Aquaspirillum dispar, Bacillus cereus/thuringiensis, Brevibacterium otitidis,Klebsiella pneumoniae, Proteus penneri / vulgaris, Pseudomonas fluorescence biotype F, Pseudomonas marginalis, Pseudomonas mendonica, Pseudomonas sp. , and Pseudomonas viridilivida) and five yeast cultures (Candida boidini, Candida memodendra, Candida mogii, Pichia haplophia, and Sacharomyces ludwigii)were isolated from OMW, purified and reused in OMW aerobic bio-treatment. After four days of aerobic small lab scale fermentation, a 68.9 % of BOD and a 67.8 % of COD reduction was achieved. A 69.3 % of BOD and a 68.4 % COD reduction was obtained during eight days of intermediate scale bio-treatment. After fourteen days of pilot scale bio-treatment, a 69.1 % reduction was recorded in BOD values and a 68.2 % reduction in COD values. Finally, a 71.0 % BOD and a 63.9 % COD reduction was observed after thirty-one days of industrial scale bio-treatment. Anaerobic OMW experimental lab scale bio-treatment using omasomal juice as experimental inoculum achieved a reduction of 67.8 % BOD and 66.6 % COD with 6.1 litres of methane production after six weeks of incubation. Combining aerobic OMW bio-treatment with anaerobic treatment, 74.3 % of BOD and 73.2 % of COD reduction were reached. Above mentioned OMW bio-treatment processes, developed at the LAU Biotechnology Labs, achieved acceptable BOD and COD reduction rates, are low cost and suitable to be applied in small rural olive mills in Lebanon and in the Middle East.
1 Introduction
In the Mediterranean basin and since early civilizations, olive trees were valued as symbols of wisdom, peace, abundance and glory.
The olive tree is most adapted to the Mediterranean climate and is one of its characteristic species (Figure 1). The Mediterranean region being poor in water resources, rendered the olive tree to become an economically beneficial target. Olive trees depend only on rainfall and don't need irrigation.
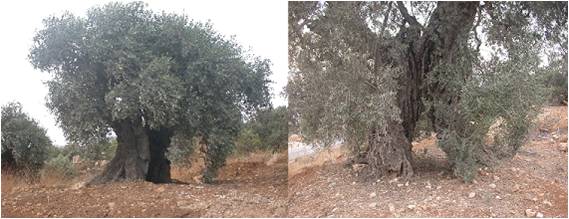
Figure 1: Hundreds years old olive trees (Hasbaya, South of Lebanon). (Microsoft Corporation, 2005)
On the other hand, olive oil extraction is one of the most polluting agro-industrial sectors world-wide due to the formation of the Olive Mill Wastewater (OMW). It is one of the two by-products obtained from olive oil extraction (Papadimitriou et al., 1997), known in Lebanon as Zibar.
2 Objectives of Study
The aim of this study is to improve Zibar treatment and bioremediation employing low cost technologies at laboratory and semi industrial scales, the objectives are:
- Isolation, purification, identification and development of microbial cultures used in OMW biotreatment.
- Trials to reduce OMW organic content thus minimizing its environmental threats.
- Construction and operation of experimental aerobic and anaerobic treatment facilities at LAU Byblos campus and in Hasbaya.
- Production of new useful and non-harmful products from the OMW biotreatment such as biogas and agricultural fertilizer.
3 Olive Mill Wastewater (OMW)
OMW accounts for up to 50 % (v/v) of the total olive oil mill output, while the olive oil accounts for 20 % (v/v), and the remaining 30 % (v/v) are the solid residue (Pérez et al., 1998; Baccari et al., 1996; Hamdi et al., 1991) known in Lebanon as Jift. Over 7.3 million tons per year (t/y) of OMW is generated worldwide, coming from the extraction of around 1.825 million tons per year of olive oil from 9.7 million tons of olives (Feria, 2000). Many studies report that OMW is a major pollutant to surface and ground water resources in the Mediterranean basin because it is one of the least biodegradable natural compounds due to its high phytotoxic phenols content, colored organic substances and high organic matter concentration (Al Khudari, et al, 2004; Saez et al., 1992; Paredes et al., 1986;). OMW is a strongly smelling phytotoxic waste known for its antimicrobial activity (Tuncel & Nergiz, 1993; Pérez et al., 1992). General approximated constituents of the OMW could be established (Table 1) where each constituent alone has a negative environmental impact if disposed without treatment with the phenolic OMW being the most polluting constituents (Ragazzi & Veronese, 1989). OMW Biological Oxygen Demand (BOD) could reach values as high as 100 g/l while the Chemical Oxygen Demand (COD) around 200 g/l (Ubay & Ozturk, 1997). The environmental problems linked to OMW are not confined to water, but some phytotoxic effects were also observed especially on plants germination and premature fall of the fruit and vegetables senescence (Feria, 2000).
Recently new measures were implemented with many chemical, biological and physical methods being suggested due to the great failure of the landspreading and lagooning methods (Hoyos et al., 2002); the yearly increase in OMW productions and the increase in olive oil produtions. However, there is no practical solution to the Zibar problem yet. Each olive mill may adopt a different system of waste treatment. The most adopted solutions include: adsorption, aerobic treatment, anaerobic treatment, composting, decolorisation, drying or evaporation, electrolysis, filtration, membrane filtration, ultrafiltration, precipitation or flocculation, lagooning, thermal treatment, treatment by fungi and wet oxidation; and these could be used seperately or in combination (Improlive project A2, 2000).
3.1 Distribution of Olive Oil Production
Olive oil production is almost concentrated in the Mediterranean basin (Ettayebi et al., 2003). Regions dominated by a Mediterranean climate such as USA (California), South Africa (Cape), and some regions in Mexico, Argentina, and Australia are also involved in olive oil production (FAOSTAT, 2003). According to the UNCTAD (United Nation Convention on Trade and Development) records based on data from FAOSTAT (Food and Agriculture Organization Statistic), eight countries were found to produce 96 % of the world olive oil in 2003 with the rest of the world contributing to the remaining 4 % as shown in Figure 2 (FAOSTAT, 2003).
Figure 2: Main olive oil producing countries in 2003 and the evolution of world production during the last ten years (FAOSTAT, 2003).
Lebanon is one of the small olive oil producers. According to the FAOSTAT (Food and Agriculture Organization Statistics) database, Lebanon produces around 6,000 tons per year of olive oil (Improlive project A2, 2000). The sector is mostly family owned and inherited from generation to generation as is the case with other Mediterranean countries; many Lebanese depend fully or partially on the income they generate from the olive oil industry; however, what distinguishes Lebanon from other countries is the total absence of governmental supervision. Strict laws are not available to protect the environment from the OMW damages and if they exist are not implemented (Hashwa, 2003). It is estimated that around 1.6 million liters of Zibar (BOD about 50,000 mg/l) is produced in Lebanon per year and disposed directly in the areas surrounding the olive mills in digs, wells, rivers, lakes, and valleys (Al-Khudary et al., 2004). However, all alternatives used will lead to the seepage of OMW into the underground water table or other water sources (Hashwa, 2003).
Despite the existing environmental laws and regulations, disposal of Zibar into the nearest lands, streams and rivers by many olive oil mills in Lebanon, is still being practiced to avoid bearing additional economic costs (Figure 3).
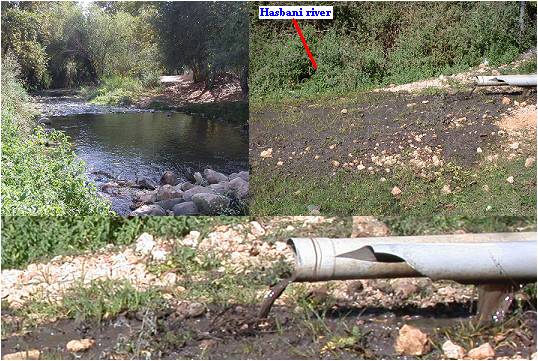
Figure 3: Crude Zibar discharge into the Hasbani River by a local olive mill (Hasbaya, Zibar project, LAU).
3.2 Components of OMW
OMW constituents are similar in all olive oil mill production, but they differ in concentrations. Approximated constituents of the OMW and their relative concentrations are given in Table 1.
Table 1: Characteristics and composition of OMW
(Skerratt & Ammar, 1999; Lopez, 1992)
OMW characteristic | Value |
Color | Intensive violet-dark brown up to black |
Odor | Strong specific olive oil smell, foetid smell |
pH | 4.5 - 6.0 |
Water Content | 83 - 92 % |
Organic and Volatile Material | 7 - 15 % |
Mineral Solids | 1 - 2 % |
Residual Oil | 0.3 - 10.0 % |
Total Sugars | 2 - 8 % |
Reducing Sugars | 1 - 8 % |
Polyalcohols | 1.0 - 1.5 % |
Protein | 0.5 - 7.5 % |
Pectins and Tannins | 1.0 - 1.5 % |
Phenols | 17 % |
Suspended Solids | 5 - 35 g/l |
BOD5 | 65 - 70 g/l |
COD | 40 - 200 g/l |
Each constituent of the OMW alone presents a threat to the environment and when combined together the threat is amplified. Tannins for example, that come from the olive skin, are not harmful for humans, animals or plants; however, they add a dark black-brown dye to the water affecting light penetration. As a consequence, photosynthesis of many aquatic plants is stopped and the vision of many aquatic animals is weakened, leading to inability to locate their food sources (Improlive project A1, 2000).
As for the soil, OMW contains many acids, minerals and organics that could destroy the cation exchange capacity of the soil. This inhibits the growth of microorganisms, the soil-air- and the air-water balance and consequently reduces the soil fertility (Improlive project A2, 2000).
4 Methodologies, applications and results
Zibar contains high concentration of organic materials that decreases the Dissolved Oxygen (DO) level in the Zibar solution. The Biological Oxygen Demand (BOD5) which measures the complete organic matter oxidation during five days was conducted on diluted samples (dilution with tap water) (dilution will enable the organic matter, present in Zibar solution, to be fully oxidized even with little oxygen concentration; also dilution is needed in order to prevent the anaerobic status of the Zibar sample during the five days incubation period; if aerobic respiration is interrupted no useful information will be gained from the BOD measurement) (Fox, 2000; Sawyer et al., 2003).
In the case of Chemical Oxygen Demand (COD) dilution is made so that the amounts of chemicals are sufficient to oxidize all the organic matters (and inorganic matter), otherwise over range values will be given by the spectrophotometer reader.
BOD, COD, and pH were measured to evaluate OMW changes before, during and after the biodegrading process. Temperature and DO were monitored to ensure optimal growth conditions for the microbial cultures.
4.1 Pilot scale aerobic treatment
The pilot plant was a 5,000 l-tank holding a volume of around 4,000 l, supplied by Nassar Technology Group (NTG), Lebanon, used normally for domestic wastewater package treatment and adapted for Zibar biotreatment (Figure 4).
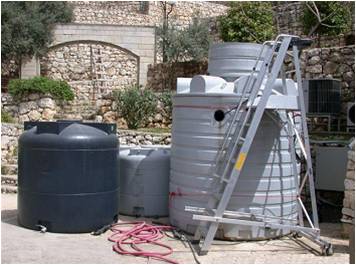
Figure 4: Aerobic OMW treatment plant (LAU, Byblos Campus, Lebanon).
The pilot tank (Figure 4), located at the LAU campus, comprised four interconnected compartments that allow continuous flow, aeration and/or sedimentation of circulating fluid (Figure 5).
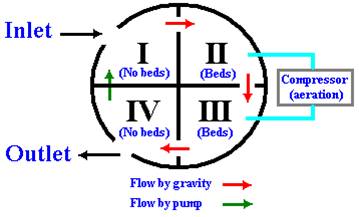
Figure 5: OMW cycle inside the pilot treatment plant at LAU, Byblos campus.
Compartment II and III contained bed columns and were aerated with an air compressor (Rietschele, Germany; 1 bar, 43.5 m3/h) housed on the top of the tank. Compartment IV contained a submersible pump, while compartment I was simply a Zibar holding container. Zibar was filled from the inlet of compartment I, overflowed from compartment I to II, III and then to IV (by gravity or difference in potential). The cycling was sustained by a submersible pump located in compartment IV, a conical shaped chamber designed for biomass sedimentation (Figure 5).
4.2 Industrial scale aerobic treatment
The industrial scale, located at Hasbaya, southern Lebanon, was annexed to Ziad Abou Ghyda olive oil mill, was composed of five large connected tanks (each similar in size to the pilot plant) filled with approximately 25,000 liters of crude Zibar (Figure 6).
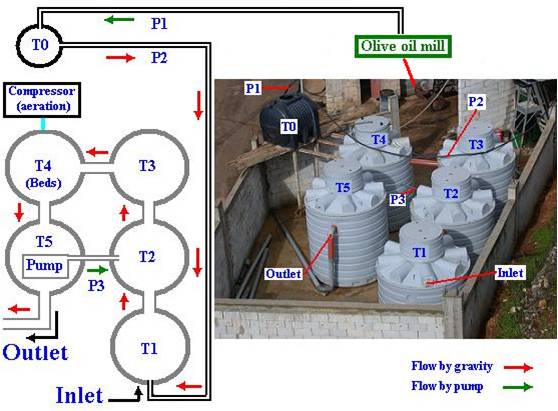
Figure 6: Industrial scale (25,000 l) treatment plant at Hasbaya (P1-3, pipes; T0 holding tank; T1-5 treatment tanks connected in series as describe in sketch)
Zibar was pumped from the nearby olive oil mill by a polyethylene pipe (P1) to an intermediate holding tank (T0). Zibar then flowed by gravity from T0 to the first tank T1 through P2 (Figure 6). T1 was used for storage and settlement. Tanks 2, 3, 4, and 5 formed a closed treatment assembly (Figure 6). The biological reactor (T4) with compressor, plastic beds, and diffusers) was inoculated with bacterial and yeast mixed cultures and was sampled at fixed time intervals. The Zibar flowed from T4 into T5, an after-clarification step tank, where a submersible pump removed treated Zibar intermittently back to T2. This closed system was kept running for about 4 weeks. The treated Zibar was discharged out of T5 sub-surface outlet into the neighboring Hasbani River (Figure 3). Zibar sludge sedimentation occurred inTanks T2 and T3 served as sedimentation tanks allowing the partially clarified Zibar to reach the aerated Bioreactor tank (T4). T4 was inoculated with a freshly prepared mixed yeast and bacterial culture transported upon need from the 80 km distant LAU campus. The starter culture (inoculum) reached about 5 % (v/v) of the total treated volume. Zibar samples were taken from T4 and occasionally from all other tanks on a weekly basis between October to March and were analyzed at LAU labs for Zibar biodegradation as indicated by changes in BOD5, COD, as well as other parameters such as DO, pH, and temperature.
4.3 Lab scale anaerobic OMW treatment
Twenty eight 250 ml Erlenmeyer anaerobic flasks were filled with 200 ml of four different mixtures (seven flasks for each solution) (Figure 7). The four sets of solutions were as follows:
- - 4,000 ml of crude Zibar, 200 ml of basal medium Demirer and Speece (1998), and 200 ml of sludge (old OMW sludge; the OMW was preserved in a closed large PVC plastic tank for months allowing natural biodegradations) .
- - 4,000 ml of treated Zibar, 200 ml of basal medium, and 200 ml of sludge (same as before).
- - 4,000 ml of 100% crude Zibar, 200 ml of basal medium, and 200 ml of omasomal juice (cows' third stomach).
- - 4,000 ml of 50 % crude Zibar, 200 ml of basal medium, and 200 ml of omasomal juice.
A control solution was also included to study the BOD base value to determine the possible omasomal juice activity (on the total BOD of the solution).
A 250 ml Erlenmeyer flask containing 200 ml mixture of 90 % water (instead of Zibar), 5 % omasomal juice, and 5 % basal medium were prepared. The BOD5 of the solution was equal to 178 mg/l.
One of the seven tubes from each set was connected to an anaerobic system for methane gas collection (Figure 16-A). The other six were caped with a rubber with a plastic tube (a pipette cut from both sides) penetrating the middle of the rubber being linked to a silicon tube (Figure 16-B). The top of the tube was submersed into water to minimize the impact of pressure coming from gases produced during anaerobic Zibar biodegradation (Figure 16-C). BOD, COD, and pH were measured on a weekly basis. The flasks were incubated at 37 oC in a waterbath.
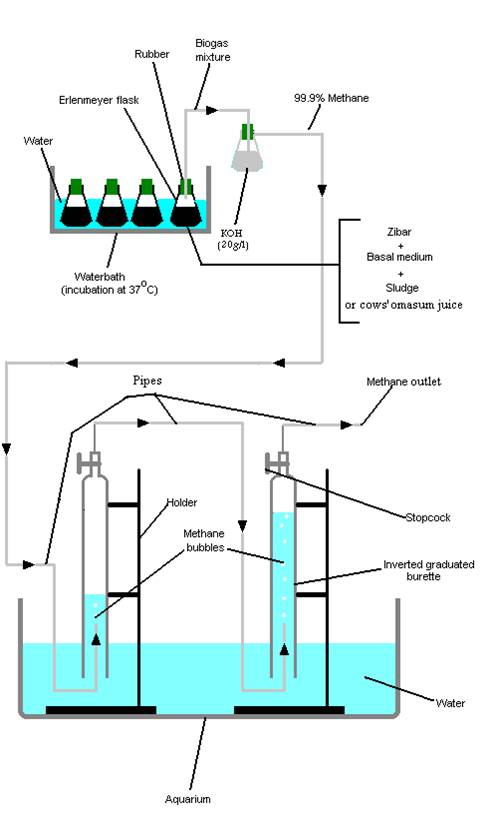
Figure 7: Sketch of the anaerobic system used for methane collection.
The produced gas mixture from the six flasks (containing the same solution) was released as bubbles and not collected, while the gas mixture of the seventh flask was collected in a 2 l Erlenmeyer flask containing a solution of 20 g/l of KOH (Figure 7). The expected gas mixture (H2, H2S, CH4, CO, CO2, N2, and H2O) would dissolve in KOH, while the biogas mixture containing 99 % methane (Demirer and Speece, 1998) was collected into inverted graduated burettes to measure the produced gas volume.
The methane gas volume was measured with respect to the volume of water displaced inside the inverted burettes. Each time the maximal volume exceeded the limiting volume of the burettes the gases were removed using a suction pump. The system was properly monitored for any gas leakage over a 3 weeks period (Figure 7).
The whole system was sealed to create anaerobic conditions with Elast Sealant (Acetoxy silicone, SABER Industrial).
RESULTS
4.2.2- Aerobic biodegradation of 100 % (v/v) crude Zibar with added aerobic microbial cultures
In the second experimental trial, aerobic microbial cultures were added to 100 % (v/v) of fresh crude Zibar.
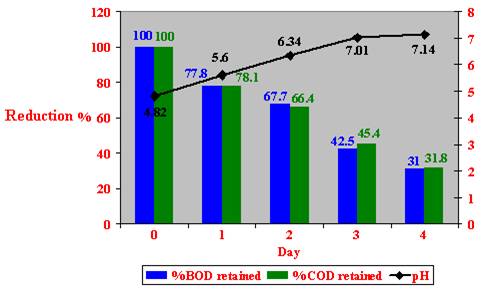
Figure 25: 100 % (v/v) crude Zibar inoculated with aerobic microbial cultures in experimental Lab scale.
A maximum of 69 % reduction in BOD and 68.9 % in COD values, and an increase of 2.32 in the pH of 100 % (v/v) crude Zibar were reported, when inoculated with aerobic microbial cultures after 4 days of incubation (Figure 25).
4.3.2- Biodegradation of 100 % (v/v) crude Zibar inoculated with aerobic microbial cultures in intermediate scale
The biodegradation potential of 100 % crude OMW was determined after 8 days of incubation with the prepared microbial cultures.
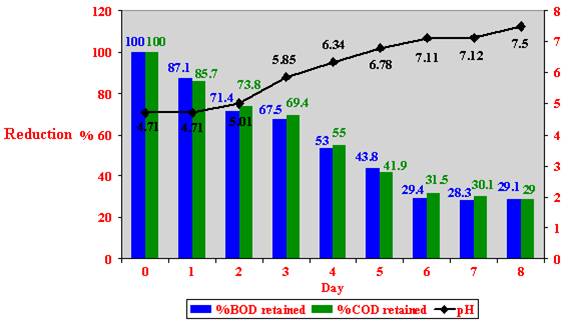
Figure 29: Biodegradation of 100 % (v/v) crude Zibar inoculated with microbial cultures in aerobic intermediate scale.
A maximum of 70.6 % reduction in BOD and 68.5 % in COD values, and an increase of 2.79 in the pH after 6 days of inoculation of a 100 % (v/v) crude Zibar inoculated with prepared microbial cultures were detected (Figure 29).
4.4.1- Biodegradation of 100 % (v/v) crude Zibar inoculated with aerobic microbial cultures in a pilot plant
In the first trial a 100 % (v/v) crude Zibar was used with the addition of aerobic microbial cultures.
A maximum of 69.6 % reduction in BOD and 68.3 % in COD values, and an increase of 2.59 in the pH after 14 days of incubation were detected (Figure 31).
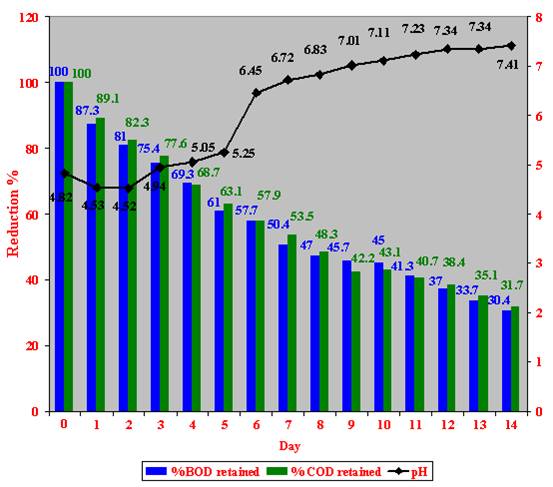
Figure 31: Fermentation trials with 100 % (v/v) crude Zibar inoculated with microbial cultures in aerobic pilot plant.
4.5- Aerobic industrial scale OMW treatment
The temperature outside the reactor ranged between 14.5 to 31.5 oC. Samples were collected on days 6, 10, 13, 20, 23, 27, and 31 (after incubation) to test for BOD, COD and pH. Unlike the other biotreatment scales, experiments on the industrial scale were performed only once (due to technical problems) over a period of 31 days (between December and January), where 100 % (v/v) of crude Zibar was used directly from the mill (Ziad Abou Ghyda olive oil mill) along with lab prepared microbial cultures.
After 31 days of fermentation, a maximum decrease of 71 % in the BOD and 63.6 % in the COD values, and 0.93 increases in the pH were detected (Figure 33).
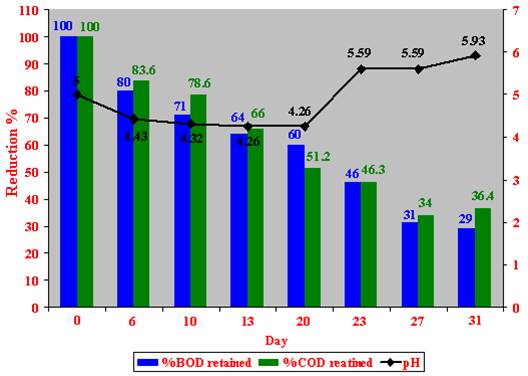
Figure 33: Industrial scale aerobic treatment of OMW (Hasbaya).
4.6- Anaerobic OMW treatment
The biogas (methane) production under anaerobic conditions was studied during an alternative OMW biotreatment process. The formed gas mixture (CO2, CH4, and H2S) was trapped first in a KOH solution (20 g/l) and released inside the inverted burettes (Figure 34) [the released gas composition was not determined and was assumed to be 99 % methane according to Ergüder et al. (1999)].
4.6.3- Anaerobic treatment of 100 % (v/v) crude Zibar using omasomal juice as inoculum
A 100 % (v/v) of crude Zibar was inoculated with omasomal juice and was anaerobically than well as aerobically biotreated.
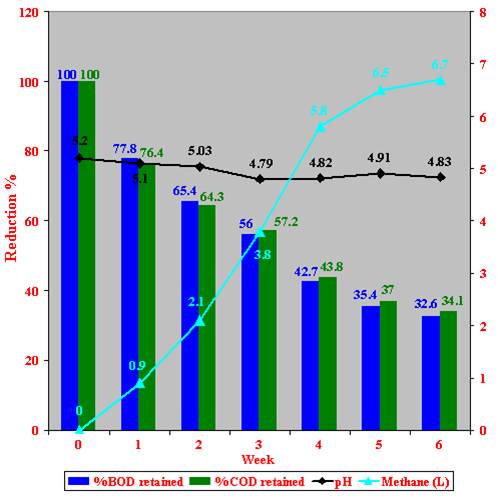
Figure 37: Anaerobic 100 % (v/v) Zibar treatment of a crude Zibar using omasomal juice as inoculum.
A maximum reduction of 67.4 % in BOD and 65.9 % in COD values, and a decrease of 0.37 in the pH were measured after 6 weeks of incubation of 100 % (v/v) crude Zibar with anaerobic bacterial cultures (omasomal juice) in experimental scale was shown, with the production of 6.7 l per six weeks of methane (Figure 37).
The anaerobically treated 100 % (v/v) crude Zibar was again aerobically biotreated using the same microbial cultures as Results 4.1 and under the same conditions as that of aerobic experimental lab scale (Results 4.2.2).
DISCUSSION
The uncontrolled disposals of Olive oil Mill Wastewater in the environment represent a serious environmental problem. The antimicrobial activity (Capasso et al., 1995; Paixao et al., 1999), the inhibition of seed germination (Bonari et al., 1993; Perez et al., 1986), the phytotoxicity to herbage crops (Capasso et al., 1992; Tomati and Galli, 1992), and the production of stale odor by OMW have been demonstrated. OMW pollution is not limited to lands, but also could reach aquatic environments, surface and underground waters (Moreno et al., 1990; Mendia et al., 1986). Accordingly, high OMWs organic concentration and content of antimicrobial compounds, such as phenols, should be subjected to pretreatment before being discharged in the environment (Ehaliotis et al., 1999). OMW treatment can be achieved satisfactorily by various techniques. As these techniques have a high fixed and operational cost, the majority of olive mills cannot afford to adopt them leading to the spread of the pollution problems. The technical-economic problems such as the requirement of a large installation spaces, professional manpower, and high economic costs of the proposed treatment techniques are all limiting factors in the OMW treatment (Ettayebi et al., 2003).
The main objective behind this research study was to develop a low capital cost operational OMW treatment techniques that can reduce the high organic load of OMW, minimize its toxic impacts on the environment, and are suitable to be adopted by the Lebanese olive mills in order to minimize the OMW negative environmental impacts. The biological treatments or biotreatments of OMW are seen as treatments of choice, since they are low cost and are capable of converting toxic compounds to useful commercially valuable such as single-cell proteins, agricultural fertilizers, phenolic compounds, and biogas (methane) (Ettayebi et al., 2003; MINOS Project 2004). This study however, was limited to biogas production.
This research study was conducted on 3 phases to look into methods for the alleviation of the negative environmental impact associated with the discharge of OMW. The treatment methodology included: aerobic biotreatment phase, anaerobic-aerobic biotreatment and finally a decolorization phase. The aerobic phase was carried at different fermentation levels, starting with the small lab volumes and scaling them up to intermediate scale (100- 300 ml), followed by the pilot (5,000 l) and the industrial scale of 25,000 liters. All aerobic treatments require relatively simple installation and maintenance; including the industrial scale, the cost is rather low and can be afforded by small to medium sized Lebanese olive mills. In contrast the anaerobic biotreatment process, being more complex, was only experimented at a small lab scale. In the proposed aerobic treatment the OMW was assessed in terms of biodegradation, in term of BOD and COD reduction, while the released byproducts including biogas (methane) under anaerobic conditions were collected and assessed to evaluate the efficiency of the small scale anaerobic fermentation.
In order to perform the different aerobic fermentations, the following microorganisms (bacterial and yeast cultures were isolated and purified for enrichment cultures, where Zibar was the main culture medium. The microorganisms included the following bacterial strains: Aquaspirillum dispar, Bacillus cereus / thuringiensis, Brevibacterium otitidis, Klebsiella pneumoniae, Proteus penneri / vulgaris, Pseudomonas fluorescence biotype F, Pseudomonas marginalis, Pseudomonas mendonica, Pseudomonas sp., and Pseudomonas viridilivida, and yeast strains: Candida boidini, Candida mogii Candida memodendra, Pichia haplophia, and Saccharomyces ludwigii. A survey of the literature revealed very little information about OMW microbial aerobic fermentations and their related microbial involvement (Literature Review -2.7.1). The microorganisms when inoculated under the proposed conditions during aerobic OMW biodegradation (Materials and Methods -3.4.1, -3.4.2, -3.4.3, and -3.4.4) seems to satisfy the optimal conditions of the aerobic microbial mixtures, due to the biodegradation results and the ability to reisolate these microorganisms at the end of OMW aerobic biotreatment. Trials of aerobic fermentation on a lab scale with 100 % (v/v) Zibar, 100 % (v/v) Zibar inoculated with aerobic microbial cultures, 50 % (v/v) Zibar, and 50 % (v/v) Zibar inoculated with microbial cultures) during a period of 4 days, the BOD and COD reduction values were similar. They ranged between 66.1 to 71.1 % of BOD and a 64.7 to 69.1 % of COD reduction. The inoculations as well as the Zibar concentrations conditions did not affect the reduction rate. These values were relatively similar to the ones achieved by Ettayebi et al. (2003) (69.7 % COD reduction) while they were better than Mendoça et al. with 50 % COD reduction. The fact that the presence or absence of microbial inocula gave similar result emphasizing the role of the indigenous microorganisms found in OMW. The 50 % (v/v) dilution, also didn't affect microbial growth and biodegradation rate although OMW is usually characterized by toxicity toward other microorganisms (Ettayebi, 2003). For the three other performed aerobic fermentations (intermediate, pilot, and industrial scales) no published data were found to compare with, such large fermentation scale have been done only with anaerobic biotreatment. The three trials with the intermediate scale fermentation (100- 300 ml) showed relatively different results; a 68.0 to 70.6 % of BOD and a 67.9 to 68.8 % COD reduction was obtained. These values, however, were obtained during 8 days of fermentation with 100 % (v/v) crude Zibar, while 6 days were needed when 100 % (v/v) crude Zibar were inoculated with microbial cultures; and 5 days were needed to achieve these reduction when the fermentation was done with 50 % v/v crude Zibar inoculated with microbial cultures. So those results demonstrated the positive effect of microbial inoculation and dilution on the speed of the fermentation processes. In the 2 pilot plant (5000 l) fermentation trials, a 68.6 to 69.6 % reduction in BOD and a 68.0 to 68.3 % reduction in COD values were recorded. But this process required an incubation period of 14 days for the biodegradation of 100 % (v/v) crude Zibar inoculated with aerobic microbial mixed cultures, and 9 days when 50 % (v/v) crude Zibar was inoculated with the aerobic microbial cultures, thus emphasizing the dilution effect on biodegradation time. Also it is worthy to mention that the LAU Biotechnology Labs succeeded to evolve and improve the biodegradation efficiency of the pilot plant supplied by the NTG company. Biotreatment should be improved with respect to the choice of microorganism used as well as to the biotreatment conditions (Ettayebi, 2003). The NTG pilot plant was designed to work under different conditions that satisfy aerobic biodegradation of domestic wastewater. The domestic sewage had a pH ranging between 7.5 and 8 (not industrial wastewater; 2.5 ≤ pH ≤ 10) and a BOD around 0.35 g/l, while OMW pH varied between 4.5 and 6.0 and a BOD of 50 g/l (Qureshi et al., 2004; Improlive project A1, 2000; Skerratt and Ammar, 1999; and Lopez, 1992); improvement was realized on the aeration (more powerful compressor; raised 10 times) and diffuser qualities (Materials and Methods -3.4.3). During summer time the temperature inside the pilot plant exceeded the optimal temperature of the aerobic microbial culture mixture to ranges above 55 and 60 oC. Therefore the biodegradation cycle was blocked, as a result the compressor work time was reduced in order to decrease the temperature and let the cycle continues. The melting point of fats and long chain fatty acids is often well above ambient temperatures. Above their melting temperature, these substances become liquid and are more accessible to microorganisms and their lipolytic enzymes; diffusion coefficients and the solubility, of fatty acids in aqueous media increase significantly with rising temperatures allowing for a better mass transfer (Becker et al. 1999). It was worthful, at these higher temperatures, to exchange the aerobic inoculum with a thermophilic one such as Thermoactinomyces putidus(Literature Review -2.3) and to study the effects, or simply to supply the air chamber where compressor is housed with a ventilator to cool down the chamber. The industrial scale fermentation run showed a maximal decrease of 71 % of the BOD and 63.6 % in the COD values during 31 days. These are relatively acceptable values. The study was limited to one trial; as this installation which was located at Hasbaya (South Lebanon), was much more laborious to operate than all the other scales. It was not only because of the much larger OMW volume (25000 l) that are to be fermented, but mostly due to logistical problems such as the shortage in maintenance (due to the long distance between LAU campus lab and the plant location) to frequent interruption in electrical power supply that was needed for the continuous operation of the compressor and pumps. The four levels of aerobic biotreatment achieved acceptable results in BOD and COD reduction rates achieved during relatively short time. The pilot plant and industrial scale fermentations could be recommended in Lebanon and other Middle East countries as the OMW treatment of choice since they are low cost, and can be locally maintained and manufactured.
The experimental set up used in the anaerobic biotreatment was assembled from normal lab glassware to fit experimental conditions, i.e. incubation at constant temperature, anaerobic conditions, and gas collection. The anaerobic biotreatment was only conducted on the samll lab scale for technical reason. The aim of using the anaerobic technology was to produce a valuable energy source biogas. In the first and second experimental trials, old OMW sludge was used to inoculate old OMW , both anaerobically treated and untreated. No significant BOD or COD values were scored out, no significant reduation was achieved, also no meaningful methane production was observed; this could be due to that old OMW, stored for a period (before 1 year), that was already naturally anaerobically fermented during the storage period; and due to the aerobically pretrated OMW used where most OMW matter had been degraded. In a third trial using fresh 100 % (v/v) OMW, inoculated with omasomal juice as inoculum, a reduction of 67.4 % BOD and 65.9 % COD with 6.7 l per six weeks of methane production was observed; while for the fouth trial where 50 % (v/v) diluted , inoculated with omasomal juice as inoculum, 68.2 % BOD and 67.3 % COD with 5.5 per six weeks of methane production was observed; the outputs of both trials (3rd and 4th) were reexposed to additional aerobic OMW biotreatment. In the third trial a total (anaerobic then aerobic biotreatment) of 86.5 % of BOD and 84.6 % COD reduction were reached; and in the fourth one 89.7 % of BOD and 88.2 % COD reduction were yielded. According to Kamra (2005) the optimal growth for the omasomal microorganisms is 39 oC while the experimental incubation temperature was fixed at 37 oC to satisfy the conditions set by Ergüder et al. (1999) (35 ± 2 oC) for anaerobic biotreatment using old OMW sludge as inoculum; that's could explain the better results scored by Ergüder et al.'s experiment, where COD removal efficiencies ranged between 93.4 and 85.4 % compared to 65.9 to 67.3 % in the experiment, and a 11.42 ± 0.3 l of biogas compared to 5.5 to 6.7 l, during 44 days of inoculation; in addition better results were achieved by Marques (2001) with 70 to 80 % reduction in the COD values, inside an up-flow anaerobic filter during 6 to 7 days, also a removal efficiency of 80 to 85 % in COD values were yielded by Sabbah et al. (2003).
CONCLUSIONS
- This study reports that the aerobic microbial culture that was used in OMW biotreatment was constituted of the following microorganisms: ten bacterial strains: Aquaspirillum dispar, Bacillus cereus / thuringiensis, Brevibacterium otitidis, Klebsiella pneumoniae, Proteus penneri / vulgaris, Pseudomonas fluorescence biotype F, Pseudomonas marginalis,Pseudomonas mendonica, Pseudomonas sp., andPseudomonas viridilivida; and five yeast strains:Candida boidini, Candida mogii, Candida memodendra, Pichia haplophia, and Saccharomyces ludwigii.
- After four days of aerobic experimental lab scale fermentation, a 68.9 % of BOD and a 67.8 % of COD reduction was achieved.
- A 69.3 % of BOD and a 68.4 % COD reduction was obtained during eight days of intermediate scale biotreatment.
- After fourteen days of pilot scale biotreatment, a 69.1 % reduction was recorded in BOD values and a 68.2 % reduction in COD values.
- A 71.0 % BOD and a 63.9 % COD reduction was observed after thirty-one days of industrial scale biotreatment.
- An additional reduction of 18.8 % in BOD and 21.5 % in COD values with 0.7 l of methane production, after 7 weeks of inoculation with OMW sludge, was reported.
- Anaerobic OMW experimental lab scale biotreatment using omasomal juice as experimental inoculum culture achieved a reduction of 67.8 % BOD and 66.6 % COD with 6.1 l of methane production after six weeks of incubation.
- Combining aerobic OMW biotreatment with anaerobic treatment, 74.3 % of BOD and 73.2 % of COD reduction were reached.
Acknowledgement: The authors thank Mercy Corps, Lebanon and LAU for a research grant in 2003-4. We thank N. Abi Chedid for skilful help
References
Al-Khudary, R., Hashwa, F., & Mroueh, M. (2004). A novel olive oil degrading thermoactinomyces species with a high extremely thermostable activity. Engineering in Life Sciences, 1, 78-82.
Baccari, M., Bonemazzi, E., Majone, M., & Riccardi, M. (1996). Interactions between acidogenesis and methanogenesis in the anaerobic treatment of olive mill effluents. Water Research, 30, 183-189.
Becker, P., Koster, D., Popov, M., Markosian, S., Antranikian, G., & Markl, H. (1999). The biodegradation of olive oil and the treatment of lipid-rich wool scouring wastewater under aerobic thermophilic conditions. Food Science and Technology, 33, 653-660.
Bonari, E., Marcchia, M., & Ceccarini, L. (1993). The waste waters from olive oil extraction: their influence on the germinative characteristics of some cultivated and weed species. Agriculture and Medicine, 123, 273-280.
Capasso, R., Cristinzio, G., Evidente, A., & Scognamiglio, F. (1992). Isolation, spectroscopy and selective phytotoxic effects of polyphenols from vegetablewaste waters.Phytochemistry, 31, 4125-4128.
Capasso, R., Evidente, A., Schivo, L., Orru, G., Marcialis, M., & Cristinzio, G. (1995). Antibacterial polyphenols from oilve mill waste waters. Journal of Applied Bacteriology, 79, 393-398.
Demirer, G. N., & Speece, R. E. (1998). Anaerobic biotransformation of four 3-carbon compounds (acrolein, acrylic acid, allyl alcohol and n-propanol) in UASB reactors. Water Research, 32 (3), 747-759.
Ehaliotis, C., Papadopoulou, K., Kotsou, M., Mari, I. & Balis, C. (1999).Adaptation and population dynamics ofAzotobacter vinelandii during aerobic biological treatment of olive-mill wastewater. Microbiology Ecology, 30 (4), 301-311.
Ergüder, T. H., Güven, E., & Demirer G. N. (1999). Anaerobic treatment of olive mill wastes in batch reactors. Report, ATD Environmental Technologies Ltd. and the State Planning Organization of the Republic of Turkey, Ankara, Turkey.
Ettayebi, K., Errachidi, F., Jamai, L., Tahri-Jouti, M., Khalid Sendide, K., & Ettayebi, M. (2003). Biodegradation of polyphenols with immobilized Candida tropicalis under metabolic induction. Microbiology letters, 223(2), 215-219.
FAOSTAT database (2003). Retrieved April 26, 2004, from:http://apps.fao.org/default.htm.
Feria, A.L. (2000). The generated situation by the OMW in Andalusia. Actas/ proceeding- Workshop Improlive Anexo A1/ Annex A1 Spain, 55-63.
Hamdi, M., Khadir, A., & Garcia, J.L. (1991). The use of aspergillus niger for the bioconversion of olive mill waste waters. Applied and Environmental Microbiology, 34, 828-831.
Hashwa, F. (2003). Biotreatment of olive mills liquid waste (Zibar) in Hasbaya. Report, Mercy Corps/ USAID, Beirut, Lebanon.
Hoyos, S., Neito, L., Rubio, F., & Cormenzana, A. (2002).Kinetics of aerobic treatment of olive oil-mill wastewater (OMW) with Aspergillus terrus. Process Biochemistry, 37, 1169-1176.
Improlive project A1 (2000). European Commission, Universidad Complutense De Madrid, Actas/ proceeding-Workshop Improlive AnexoA1/ Annex A1 2000. Final Report- Annex A1.
Improlive project A2 (2000). Improvements of treatment and validation of the liquid solid waste from the two phase oil extraction. EC report (Fair CT96- 1420) Final Report- Annex A2.
Kamra, D. N. (2005). Rumen microbial ecosystem. Current Science, 89 , 1-10.
Lopez, R. (1992). Land treatment of liquid wastes from the olive oil industry (Alpechin). Fresenius Enviromental Bulletin, 1, 129-134.
Marques, I. P. (2001). Anaerobic digestion treatment of olive mill wastewater for effluent re-use in irrigation.Desalination, 117, 233-239.
Mendia, L., Carbone, P., Antonio, G., & Mendia, M. (1986). Treatment of olive oil waste waters. Water Sciences and Technology, 18, 125-136.
Mendoça, E., Martins, A., & Anselmo, A. M. (2004). Biodegradation of natural phenolic compounds as single and mixed substrates by Fusarium flocciferum. Electronic Journal of Biotechnology, 7, 1.
MINOS Project (2004). Process development for an integrated olive oil mill waste management recovering natural antioxidants and producing organic fertilizer. Athens, Greece: TERRA NOVA Ltd.
Mhanna, Y. E. (2006). Aerobic and anaerobic biotreatment of olive oil mill waste water. Byblos, Lebanon: Lebanese American University.
Moreno, E., Quevedo-Sarmiento, J., & Ramos-Cormenzana, A. (1990). Antibacterial activity of wastewaters from olive oil mills: Hazardous Waste Containment and Treatment. USA: Gulf Publications.
Papadimitriou, E.K., Alexiou, I.E., Chew, T.Y., Balis, C. & Stentiford, E.I. (1997). Guidelines for Biological Treatment of Vegetable Oil Extraction Wastewaters. Proceedings of the 6th IAWQ Asian WaterQual Conference in Seoul, Korea, 1, 766-773.
Paredes, M.J., Monteoliva-sanchez, M., Moreno, E., Perez, J., Ramos-Cormenzanz, A., & Marinez, J. (1986). Effect of waste waters from olive oil extraction plants on the bacterial population of soil. Chemosphere, 15, 659-664.
Paixao, S. M., Mendoça, E., Picado, A., & Anselmo, A. M. (1999). Acute toxicity evaluation of olive mill wastewaters: A comparative study of three aquatic organisms. Environmental Toxicology, 14, 263-269.
Perez, J. D., Esteban, E., Gomez, M., & Gallardolara, F. (1986). Effects of waste water from olive processing on seed germination and early plant growth of different vegetable species. Journal of Environmental Sciences and Health, 21, 349-357.
Pérez, J., De La Rubia, T., Ben Hamman, O., & Martínez, J. (1998). Phanerochaete flavido-alba laccase induction and modification of manganese peroxidase isoenzyme pattern in decolorized olive oil mill wastewaters. Applied and Environmental Microbiology, 64 (7), 2726-2729.
Pérez, J., De La Rubia, T., Moreno, J., & Martínez, J. (1992). Phenolic content and antibacterial activity of olive oil waste waters. Environmental Toxicology and Chemistry, 11, 489-495.
Qureshi, S., Richards, B. K., Steenhuis, T. S., McBride, M. B., Baveye, P., & Dousset, S. (2004). Microbial acidification and pH effects on trace element release from sewage sludge.Environmental pollution, 132, 61-71.
Ragazzi, E., & Veronese, G. (1989). The effect of oxidative coloration on the methanogenic toxicity and anaerobic biodegradability of phenols. Biological Water, 32, 210-225.
Sabbah I., Marsook, T., & Basheer, S. (2003). The effect of pretreatment on anaerobic activity of olive mill wastewater using batch and continuous systems. Process Biochemstry. Article in press, Elsevier Ltd.
Saez, L., Perez, J., & Martinez, J. (1992). Low molecular weight phenolic attenuation during simulated treatment of wastewaters from olive oil mills in evaporation ponds. Water Research, 26, 1261-1266.
Skerratt, G., & Ammar, E. (1999). The application of reedbed treatment technology to the treatment of effluents from olive oil mills. Final report, ENIS/LARSEN - Staffordshire University, UK.
Tomati, U., & Galli, E. (1992). The fertilizing value of waste waters from the olive processing industry: Humus, its structure and role in agriculture and environment. Amsterdam: Elsevier Science.
Tuncel, G., & Nergiz, C. (1993). Antimicrobial effect of some olive phenols in a laboratory medium. Letters in Applied Microbiology, 17, 300-302.
Ubay, G. & Ozturk, L. (1997). Anaerobic treatment of olive mill effluents. Water Science Technology, 35, 287-294.
LAU is AFED Academic Member. http://www.lau.edu.lb